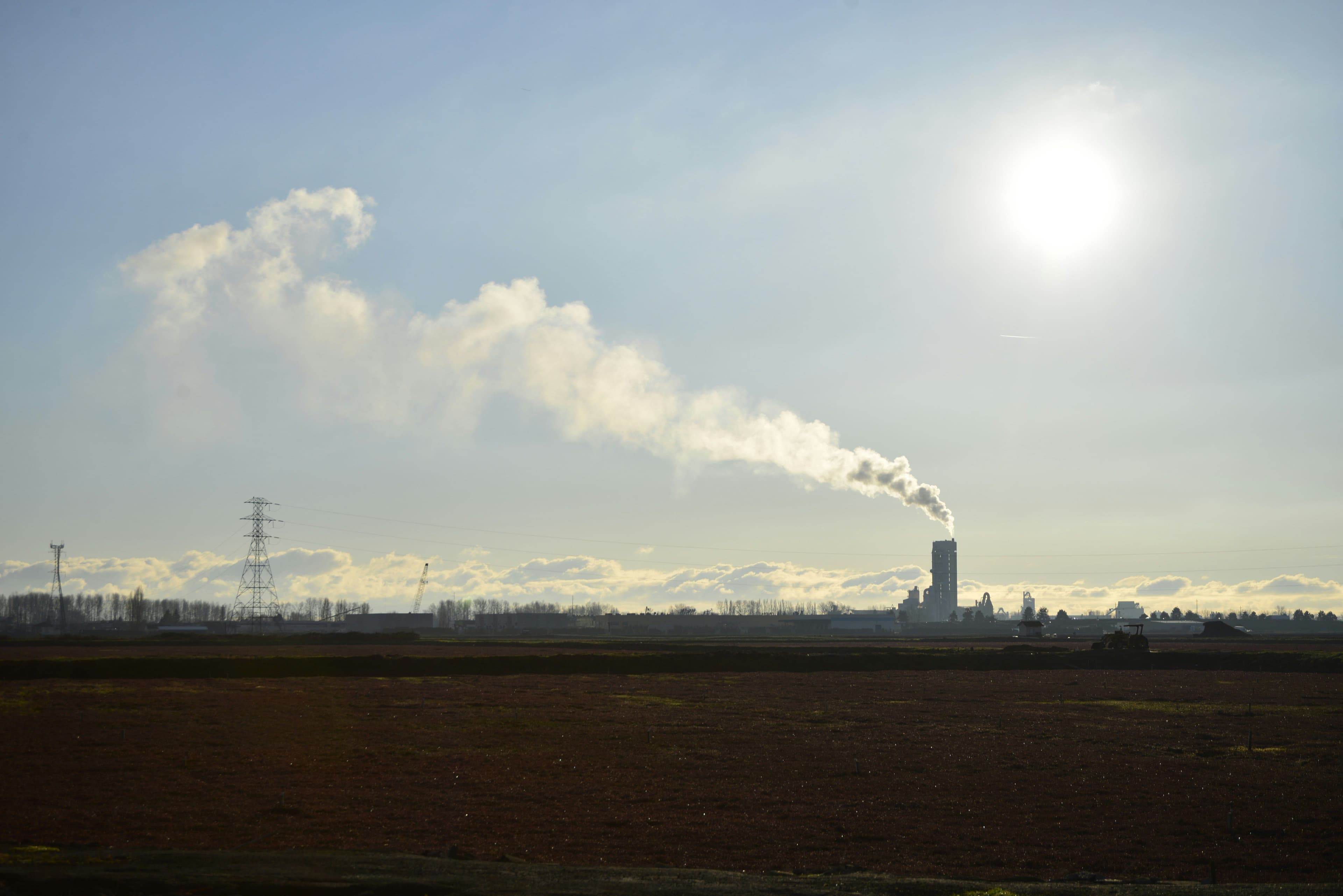
Upcycling carbon dioxide into products like e-fuels converts a harmful greenhouse gas into an economic asset. But this process has been challenging: carbon dioxide doesn’t like to change and requires a lot of energy to be transformed to new molecules. Cheap solar power however reduces the energy costs of transformation, while new innovations in synbio and electrochemical synthesis offer more efficient transformation processes. Together, this makes certain products cost-effective.
Introduction
Upcycling carbon dioxide into products like e-fuels, sustainable plastics, and high-value chemicals converts a harmful greenhouse gas into an economic asset. This process has been challenging: carbon dioxide doesn’t like to change and requires a lot of energy to be transformed to new molecules.
Roadrunner now believes new innovations will finally allow for carbon dioxide upcycling. Cheap solar power reduces the energy costs of transformation, while new innovations in synthetic biology and electrochemical synthesis offer more efficient transformation processes. Together, this makes certain products cost-effective.
We’re interested in founders, start-ups, and scientists with innovative ideas on how to move the needle in this space. Read on to learn more about the areas that are most exciting to us.
Our Core Beliefs
- Flue gas and BECCS will beat out Direct Air Capture (DAC) for carbon feedstock supply until an order of magnitude improvement in DAC technology is demonstrated.
- Cheap, abundant hydrogen fuel (H2) will accelerate any and all carbon-to-chemical processes. New markets are unlocked when hydrogen is available at ≤$1/kg H2, currently hard to achieve without heavy emissions.
- Complex & high margin chemicals must be the beachhead markets for renewable carbon products. Simple commodity chemicals — alkanes, alcohols — will struggle to achieve profitability with theoretical feedstock costs nearly matching current prices.
Topics That Excite Us
- Biological/microbial routes for chemical production. Complex, high margin chemicals are hard to make. Biology may offer more efficient routes to these products.
- Plasma-mediated processes. Plasma chemistry generates highly reactive environments where chemical reactions are driven by energetic species such as radicals, ions, and excited molecules. It may be effective for transforming highly-stable CO2. In addition, plasma can be efficiently powered by renewable electricity, unlocking green chemical processes.
- Creative end products. Can CO2 be used to create diamonds? What about dietary fats? Carbon is the basis for a number of materials, so figuring out the right end product is key.
Deep Dive
The Challenge of CO2
Transforming carbon dioxide into fuels and chemicals means competing against nature. Carbon dioxide sits at the bottom of the energy well and is very comfortable there, requiring substantial energy inputs to reduce it to a more reactive state. Gathering significant quantities of carbon dioxide is also difficult.
Real DAC technologies are only ~5% efficient, generally requiring around 400 kJ/mol/CO2 (2500 kWh/tonne CO2eq) to do the same. To put this in perspective, a coal-fired power plant produces roughly 158 kJ/mol CO2eq, while a NG fired power plant produces 396 kJ/mol CO2eq.
Until we can gather CO2 from the atmosphere more efficiently, other carbon sources for carbon-to-chemical renewable processes may create more value. One option is sourcing CO2 directly from flue gas, another is extracting CO2 from biomass.
First Principles
Roadrunner decided that to be successful, renewable carbon-based products must be cost-competitive with the fossil-fuel based versions. We can decide what products could be cost competitive by analyzing the cost of the “ingredients” of that product. Captured CO2 (or biochar C) must be hydrogenated or transformed into syngas in order to produce chemicals/fuels. There are many reaction pathways (biologic, thermal, electrochemical) to reduce CO2 to CO. For our analysis, we assumed one carbon dioxide molecule could be turned into one carbon monoxide molecule. We also assumed additional H2 would need to be added to our feedstock, as most processes use approximately 2:1 H2:CO for chemical production.
We then modeled the cost of making the final product based on the total cost of the carbon and hydrogen that would go into it, using a variety of carbon and hydrogen prices.
Targeting High-Value Products
Figure 1 illustrates this cost assessment for methane and methanol (low/low-ish margin commodity chemicals) and propylene (higher margin commodity chemical) across different CO2 and H2 prices. The Y axis represents the cost of the “ingredients” for that end product — this is the lowest possible cost for making that product if the transformation itself were free. The X axis is the cost of carbon dioxide per ton, while the black, red, and blue lines represent different costs of hydrogen. The yellow region is current carbon dioxide prices when captured from flue gas, the orange region represents carbon from bioenergy carbon capture and sequestration (BECCS), and the green region is carbon dioxide sourced from direct air capture. Grey hydrogen can currently be produced from reformers in the gulf states for about $1/kg H2 (black line) while it is envisioned that in their best case scenarios, green hydrogen and geologic hydrogen can be produced at $3/kg H2 (blue line) and $0.3/kg H2 (red line), respectively.
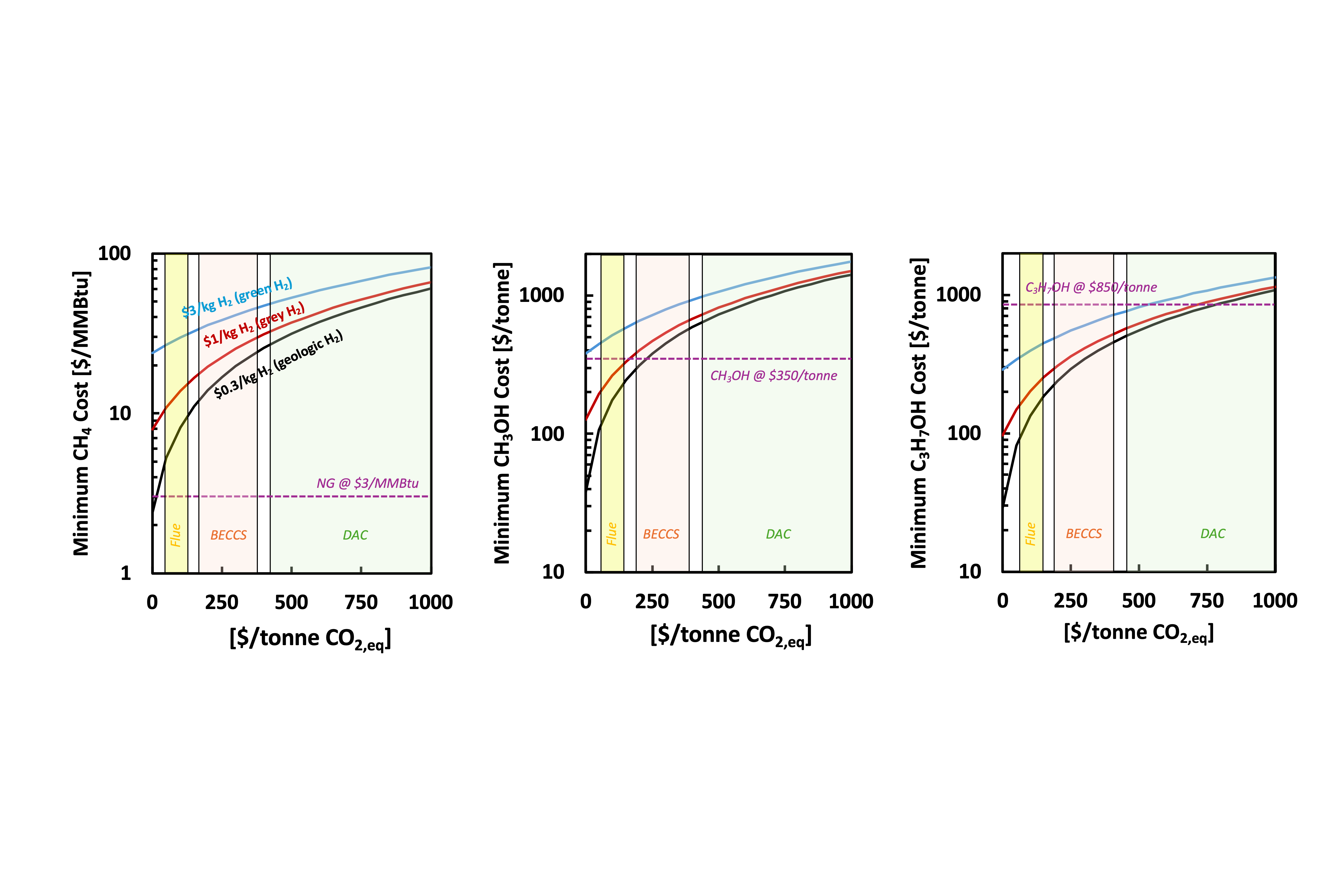
Figure 1. Minimum cost of synthetic methane (left), methanol (middle), and propylene (right) produced from CO2 as a function of CO2 cost as well as cost of hydrogen from 3 different sources: $0.3/kg H2 for geologic hydrogen (black), $1/kg H2 for grey hydrogen (red), and $3/kg H2 for green hydrogen (blue).
The takeaway is clear: products cost less when they are made from the cheapest sources of CO2 and H2. Based on this analysis, it would be difficult to make cost-competitive methane or methanol, as the market price for these materials is close to (or less than) the cost of the carbon and H2 that would go into them.
There’s no room for expenses associated with the manufacturing process. More complex chemicals, like propylene on the right, have more room to spend money on manufacturing on top of the carbon dioxide and hydrogen feedstock costs. This is because complex molecules are difficult to make, which is why they cost more today, which allows a CO2 feedstock to fit in more easily.
The highest value and margin carbon product is diamonds. Plasma grown diamond wafers make it easy to bury the costs of CO2 (See Figure 2) while providing a new technology that can potentially transform the world of computation, 5G/6G, and power electronics.
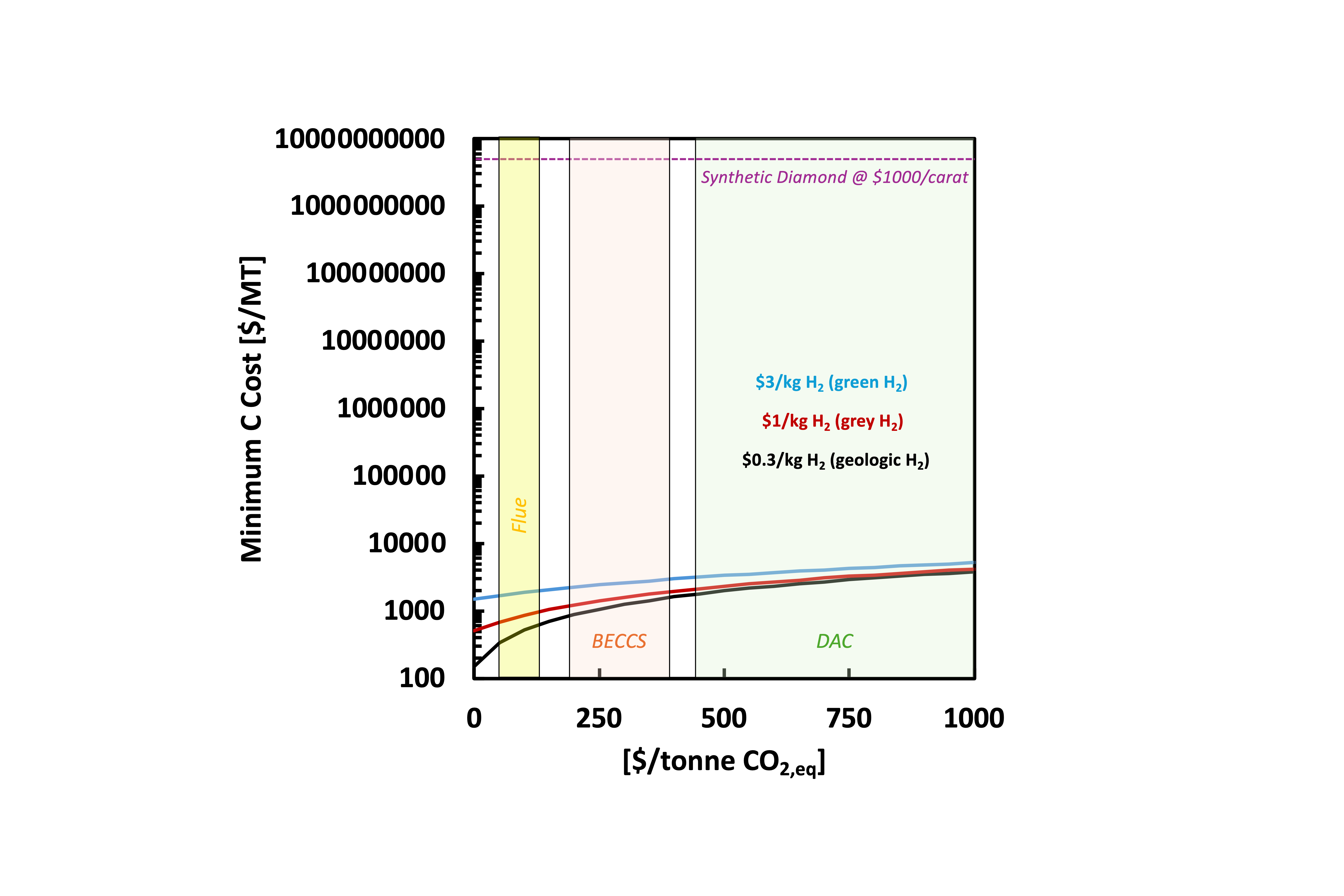
Figure 2. Minimum cost of synthetic diamond as a function of CO2 cost as well as cost of hydrogen from 3 different sources: $0.3/kg H2 for geologic hydrogen (black), $1/kg H2 for grey hydrogen (red), and $3/kg H2 for green hydrogen (blue).
Path to Product
Chemicals with low complexity (alkanes, simple alcohols/alkenes) are cheaper to make using traditional thermal routes with petrochemical feedstocks. They are so efficient that most of their cost generally comes from their feedstocks. As a chemical’s complexity increases, so does its cost (smaller markets with higher margins) and the emergence of biological/fermentation routes to efficiently achieve these complexities. This is a potential penetration point for CO2 feedstocks as most of the cost is not defined by the feedstock. Biological processes operate near room temperature keeping processes near equilibrium, leading to slower reactions but allowing for greater precision.
In essence, chemistry has a cost advantage for making simple molecules, but it vanishes quickly for making more complicated products.